Phytochrome structure/function
But it isn't easy to work plant phytochromes: they are large proteins (the functional dimer is ~250 kDa) requiring a special bilin cofactor and are thus difficult to produce heterologously in E. coli. Indeed, it is generally difficult to work with plants and other higher eukaryotes. Prokaryotes, on the other hand, are much simpler and have much smaller genomes. Because of this, one of the first genomes to be sequenced was that of the cyanobacterium Synechocystis. As a photosynthetic organism Synechocystis has many similarities to plants - but it's a lot simpler and much easier to work with. While I was a postodoc in Berlin in 1996 we discovered and characterised the first prokaryotic phytochrome (Cph1; see photo left of Cph1 in its Pr (left) and Pfr (right) photochromic states) having recognised a phytochrome-like gene in the Synechocystis genome. This discovery, which we published in Nature (Hughes et al., 1997), was quite unexpected and had a big effect on phytochrome research worldwide. No only was Cph1 the "missing link" between two-component bacterial sensory systems and plant phytochromes, it acted like a Rosetta Stone in understanding the phytochrome domain structure, making both the molecular architecture and evolutionary origin of the most important plant photoreceptor instantly clear (see our review in Plant Physiology (Hughes & Lamparter, 1999)).
It didn't stop there, though. Unlike plant phytochromes which are difficult to purify from plants themselves and tricky to obtain transgenically by overexpression in other organisms, Cph1 expresses beautifully in E. coli, allowing us to produce almost any amount of Cph1 apoprotein at very high purity. To make the functional holoprotein photoreceptor, we extract the blue bilin cofactor PCB from cyanobacteria, purify it, then add it to the apoCph1: obligingly, this then "autoassembles" to form the functional photoreceptor, holoCph1. Thus, together with our friends with the appropriate biophysical expertise as well as funding from the DFG and the Volkswagen Foundation, we were able to discover a lot about how Cph1 - and thus the phytochrome family as a whole - functions. We have published dozens of papers on this, listed here.
NMR studies
The fact that we make holoCph1 by adding the PCB chromophore to the apoprotein means that we can, for example, introduce labelled atoms into either component separately, allowing us to produce valuable material for NMR studies - in particular solid-state or "magic angle spinning" (MAS) NMR. Together with Wolfgang Gärtner at the MPI Mülheim and Jörg Matysik then at the University of Leiden (NL) and with the help of a grant from the Volkswagen Foundation, we made considerably progress using these methods. In particular, we showed that the Pr->Pfr activation mechanism involves proton migration, not deprotonation, as some had suggested (Rohmer et al. (2008) PNAS). Thiery Rohmer was also able to freeze-trap several intermediates of the relatively unknown Pfr->Pr back-reaction and analyse them via MAS NMR (Rohmer et al. (2010) JACS). His successor Chen Song was able to develop HetCor methods to allow the (unlabelled) protein matrix surrounding the (labelled) chromophore to be detected in the Pr and Pfr states (Song et al. (2011) PNAS), confirming that the primary event in Pr->Pfr photoconversion is a photoflip of ring D. Remarkably, this had become an issue on the basis of a paper prominently positioned in Nature by the Vierstra group in 2010 (see Hughes (2010) Biochem Soc Trans). We re-assessed this by MAS NMR, proving conclusively that the D-ring photoisomerizes, as had been pretty clear all along (Song et al. 2013, JBC). We were even successful with plant phytochromes: Chen used HetCor methods to investigate the 3D structure of the oat phytochrome A3 chromophore pocket in the Pr state - the first structural data for any plant phytochrome (Song et al. (2012) Mol Plant). We have now extended this study to the Pfr signaling state and the changes associated photoconversion (Song et al., (2018) Fr Pl Sci). The Matysik group is now in Leipzig, allowing the cooperation flourish further. We also cooperate with Hartmut Oschkinat at the FMP in Berlin (see our work in Sfb1078, below). This gives us access to state-of-the-art DNP enhancement (Stöppler et al. 2016, Angew. Chem.) and direct proton detection methods. Whereas it is not yet possible to solve complete protein structures via MAS NMR, the technology continues to evolve rapidly (as our publications illustrate) and the fact that it allows even quite distant atomic contacts (up to 6 Å) including hydrogen atoms / protons to be detected even in photoconversion intermediates without any need for crystallisation (see below) renders it the ideal complement to crystallographic studies. We are the only group worldwide exploiting this valuable synergy.
X-ray christalography
But, as I pointed out above, one can't (yet) solve a complete protein structure with MAS NMR. Some years ago, we developed a technology for generating holoCph1 di
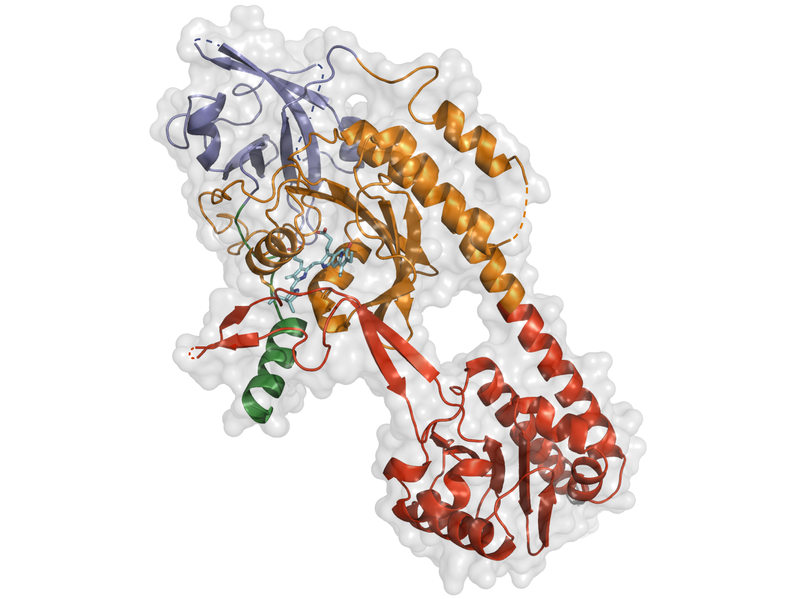
Quite unexpectedly, Kaoling found that his phyA crystals themselves could be photoconverted between Pr and Pfr without losing resolution (which he has now improved to 1.6 Å). That provides us with a unique opportunity to use the cutting-edge technology of the European X-ray free-electron laser (EuXFEL) and the T-REXX facility in Hamburg to determine the 3D structure of the protein kinetically as it absorbs light and refolds from the inactive Pr state to the Pfr signalling state. We have been granted EuXFEL beamtime and have already produced the high-quality microcrystals that the system requires. We expect a lot from this project....